Using boosters and parallel arrays – Vicor VI-J00 Family DC-DC Converters and Configurable Power Supplies User Manual
Page 16
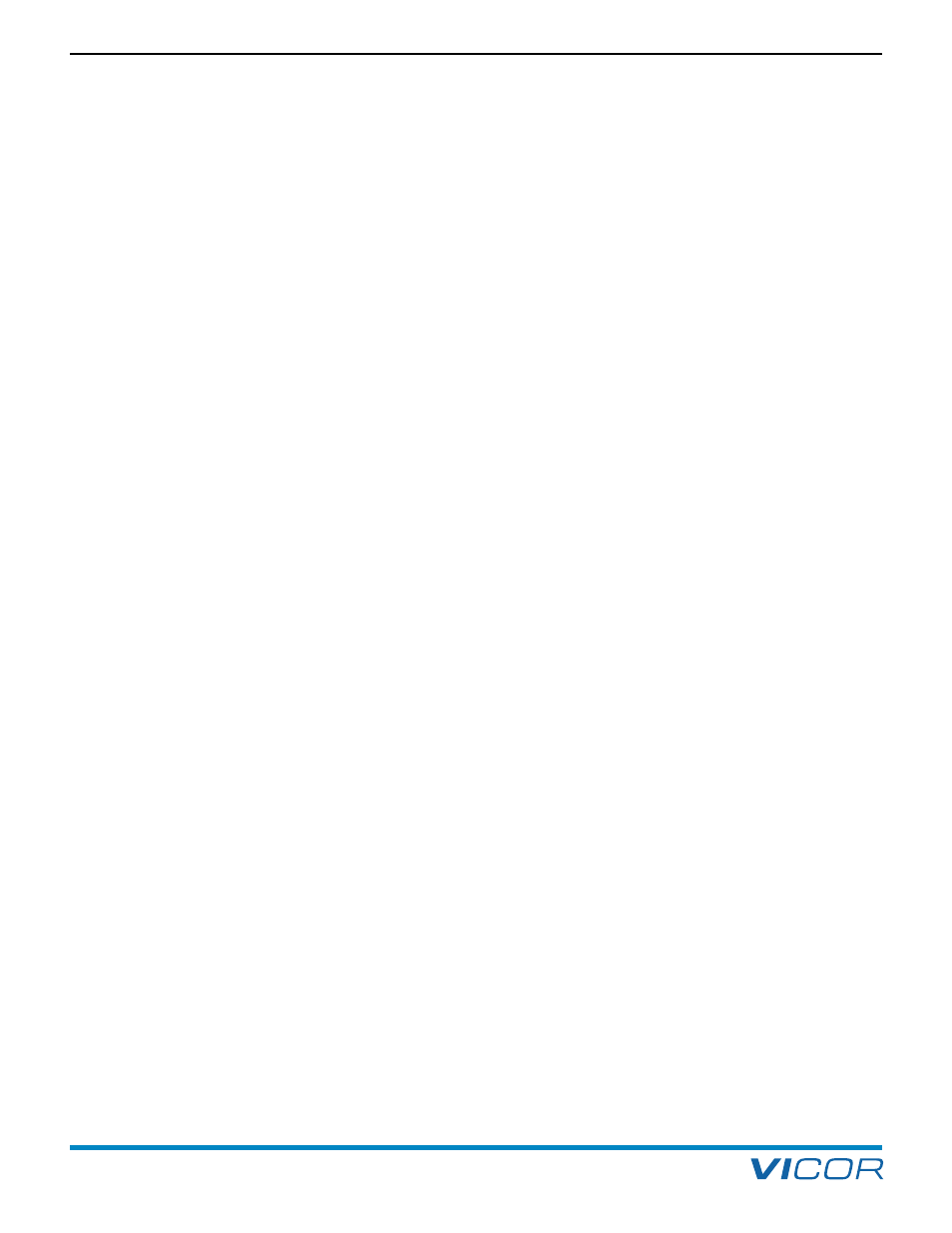
Design Guide & Applications Manual
For VI-200 and VI-J00 Family DC-DC Converters and Configurable Power Supplies
VI-200 and VI-J00 Family Design Guide
Rev 3.5
vicorpower.com
Page 15 of 98
Apps. Eng. 800 927.9474
800 735.6200
deliver some current, but the load will be shared unequally.
With built-in current limiting, one or more of the converters
will deliver current up to the current limit (generally 15 or
20% above the module’s rated maximum), while other
converters in the array supply just a fraction of load.
Consider a situation where one module in a two-module
array is providing all of the load. If it fails, the load on the
second module must go from no load to full load. During
that time, the output voltage is likely to droop temporarily.
This could result in system problems, including shutdown
or reset.
On the other hand, if both modules were sharing the load
and one failed, the surviving module would experience a
much less severe transient (one-half to full load). Also, the
output voltage would be likely to experience no more
than a slight momentary droop. The dynamic response
characteristic of all forward converters, resonant or pulse-
width modulated, is degraded when the load is stepped
from zero (no load) where the output inductor current is
discontinuous.
In the same two-module array example, the module
carrying all of the load also is generating all of the heat.
That results in a much lower mean time between failure
for that module. An often-quoted rule of thumb says that
for each 10°C increase in operating temperature, average
component life is cut in half.
In a current-sharing system, the converters or supplies all
run at the same temperature. This temperature is lower
than that of the hot-running (heavily loaded) modules in
a system without current sharing. Furthermore, same-
temperature operation means that all of the modules in
a current-sharing arrangement age equally.
Current sharing, then, is important because it improves
system performance. It optimizes transient and dynamic
response and minimizes thermal problems, which improves
reliability and helps extend the lifetimes of all of the
modules in an array. Current sharing is an essential
ingredient in most systems that use multiple power supplies
or converters to achieve higher output power or fault
tolerance.
When parallel supplies or converters are used to increase
power, current sharing is achieved through a number of
approaches. One scheme simply adds resistance in series
with the load. A more practical variant of that is the
“droop-share” method, which actively causes the output
voltage to drop in response to increasing load.
Nevertheless, the two most commonly used approaches
to paralleling converters for power expansion are Driver /
Booster arrays and analog current-sharing control. They
appear to be similar, but the implementation of each is
quite different.
Driver / Booster arrays usually contain one intelligent
module or Driver, and one or more power-train-only
modules or Boosters. Analog current-sharing control
involves paralleling two or more identical modules, each
containing intelligence.
One of the common methods of forcing load sharing in
an array of parallel converters is to sense the output
current of each converter and compare it to the average
current. Then, the output of a given converter is adjusted
so that its contribution is equal to the average. This is
usually accomplished by current-sense resistors in series
with the load, a sensing amplifier for each converter
module, and a summing amplifier. Load sharing is
accomplished by actively trimming the output voltage
using TRIM or SENSE pins.
Occasionally, a designer is tempted to avoid the expense
of a current-sense resistor by using the IR drops in the
wire as a means of sensing the current. Unfortunately,
there are a number of negative issues associated with
that idea. First of all, there’s the temperature coefficient
of copper. As the wire heats up, its resistance increases,
negating its value as a stable current-sensing device.
Second, there are oxidation and corrosion issues, which
also cause parametric changes. Consequently, a high-
precision current-sensing device, such as a precision
resistor, is a must.
The resistor values typically range from a few milliohms
up to about 100 m
Ω, depending on the power level or
current range of operation. Selecting the right value
requires a tradeoff between power dissipation and
sensitivity (signal-to-noise ratio or noise immunity). The
larger the resistor value, the better the noise immunity—
and the greater the power dissipation.
Determining the size of the resistor needed to generate a
signal above the noise can be a bit tricky. Another
potential pitfall with this (or, for that matter, any other)
approach is the need for good electrical and mechanical
design and layout. This requires adequate trace widths,
minimized trace lengths, and decoupling to reduce noise.
An experienced designer should have no difficulty with
this, but it is an area rich with opportunities for error.
The droop-share method artificially increases the output
impedance to force the currents to be equal.
It’s accomplished by injecting an error signal into the
control loop of the converter, causing the output voltage
to vary as a function of load current. As load current
increases, output voltage decreases. All of the modules
will deliver approximately the same current because they
are all being summed into one node.
If one supply is delivering more current than another
supply, its output voltage will be slightly forced down so
8. Using Boosters and Parallel Arrays